Jin-Han Xie's Research
Jin-Han Xie's Research
Jin-Han Xie's Research
Jin-Han Xie's Research
Jin-Han Xie's Research
Jin-Han Xie's Research
Jin-Han Xie's Research
Jin-Han Xie's Research
Jin-Han Xie's Research
Jin-Han Xie's Research
In oceanic fluid dynamics, my research focuses on energy transfer across scales to understand the nature of multiscale nonlinear interactions. These researches provided observational evidence of simultaneous oceanic kinetic energy transfer to both large and small scales, proposed a wave-mean interaction mechanism to explain this bidirectional transfer, and studied the impact of wave-induced downscale energy flux on jet formation. In addition, it is shown that, apart from waves, small-scale topography can also lead to bidirectional energy transfer of the geostrophic turbulence, which is traditionally believed to transfer energy upscales. These results enhance our understanding of ocean energetics and provide a more comprehensive picture of the complex nonlinear interactions within geophysical fluid systems due to rotation and stratification. This research was funded by the National Natural Science Foundation of China under the grant “Theory for rotating stratified turbulence based on wave-mean flow decomposition” with NO. 92052102.
Evidence for bidirectional oceanic energy transfer
The ocean’s turbulent energy cycle has a paradox: due to rotation, large-scale oceanic motions transfer kinetic energy to large scales; however, energy transfer to small scales is required for dissipation. It has been hypothesized, using simulations, that fronts, waves, and other turbulent structures can produce a downscale transfer of kinetic energy toward dissipation scales. However, the coexistence of upscale and downscale energy transfer has never been observed. Based on the turbulence structure-function theory beyond inertial range, we present the first evidence of a dual kinetic energy transfer in the ocean by analyzing the drifter data in the Gulf of Mexico [Balwada et al., 2022, Sci. Adv.]. Figure below shows the estimates of spectral flux from the third-order structure function for the LAgrangian Submesoscale ExpeRiment (LASER) experiment. The method of analyzing measured data using turbulent structure-function theory can also be applied to other systems, such as solar wind, and be applied to potential energy and materials, e.g., CO2 and pollution.
(Work with Dr. Dhruv Balwada, Raffaele Marino and Fabio Feraco.)
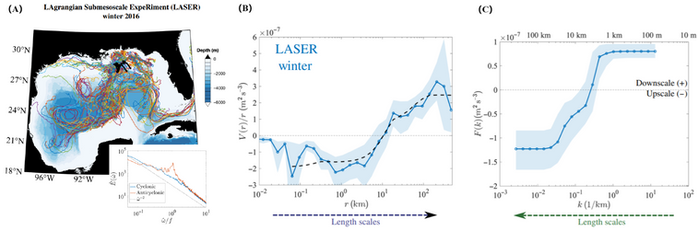
Figure: (A) Illustration of the LASER drifter paths and frequency spectrum. (B) Thenormalized third-order structure function (blue curve and markers) and the theoreticalexpression (black dashed curve). (C) The spectral energy flux.
Bidirectional energy transfer through wave-mean flow interaction
To understand the mechanism behind oceanic bidirectional energy flux, this research studied a model that captures the interaction between near-inertial waves and quasi-geostrophic mean flow in the turbulence regime. Theoretically, the conservation of energy, potential enstrophy and wave action implies the change of direction and magnitude of energy flux with varying relative strength between near-inertial wave and mean flow, leading to the bidirectional energy flux [Xie, 2020, J. Fluid Mech.] (cf. Figure on the right). We name this mechanism as the catalytic wave induction mechanism because the near-inertial wave induces a downscale energy flux of the mean flow without energy conversion between these two flow components, and it differs from the classic stimulated loss of balance mechanism where energy transfers from mean flow to waves and finally dissipates at small scales. The newly found mechanism sheds light on the bidirectional energy transfer in oceanic systems and serves as a potential explanation for the puzzle of mesoscale eddy energy balance, advancing our understanding of complex oceanic processes. For example, the catalytic wave induction mechanism implies that internal waves weaken jet formation in planet flows [Zhang and Xie, 2023, J. Fluid Mech.].
(Work with Dr. Lin-Fan Zhang)
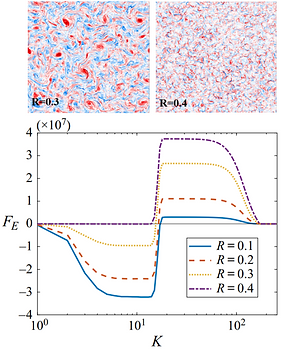
Figure 2: Mean vorticity field and spectral energy flux in model coupling near-inertial waves and quasigeostrophic mean flow with changing relative wave strength R.
Topography-induced bidirectional energy transfer in geostrophic turbulence
Seafloor topography is essential for oceanic fluid dynamics from many perspectives. This work studied the impact of small-scale topography on the dynamics of quasi-geostrophic turbulence and found that small-amplitude topography extends the range of upscale energy flux and enhances condensation, contradicting the common understanding of topographic damping (Figure on the right). Topography with an intermediate magnitude leads to bidirectional energy transfer, and there exists a critical topography magnitude of second-order phase transition, beyond which energy only transfers downscale. The discovery of counterintuitive topography-enhanced energy flux by small-scale topography, which is hard to resolve in numerical simulations, and its critical phenomenon provide a more comprehensive understanding of nonlinear oceanic fluid dynamics and
bring new challenges to topography parameterization in ocean models.
(Work with Dr. Lin-Fan Zhang)
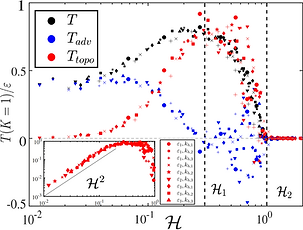
Figure: Anomalous upscale energy flux induced by the small-scaletopography shown by the incrementof Ttopo as the normalized topography height H increases when H is small.